Abstract
The coronavirus disease 2019 (COVID-19) is an unprecedented pandemic that has severely impacted global public health and the economy. Hydroxychloroquine administered orally to COVID-19 patients was ineffective, but its antiviral and anti-inflammatory actions were observed in vitro. The lack of efficacy in vivo could be due to the inefficiency of the oral route in attaining high drug concentration in the lungs. Delivering hydroxychloroquine by inhalation may be a promising alternative for direct targeting with minimal systemic exposure. This paper reports on the characterisation of isotonic, pH-neutral hydroxychloroquine sulphate (HCQS) solutions for nebulisation for COVID-19. They can be prepared, sterilised, and nebulised for testing as an investigational new drug for treating this infection. The 20, 50, and 100 mg/mL HCQS solutions were stable for at least 15 days without refrigeration when stored in darkness. They were atomised from Aerogen Solo Ultra vibrating mesh nebulisers (1 mL of each of the three concentrations and, in addition, 1.5 mL of 100 mg/mL) to form droplets having a median volumetric diameter of 4.3–5.2 µm, with about 50–60% of the aerosol by volume < 5 µm. The aerosol droplet size decreased (from 4.95 to 4.34 µm) with increasing drug concentration (from 20 to 100 mg/mL). As the drug concentration and liquid volume increased, the nebulisation duration increased from 3 to 11 min. The emitted doses ranged from 9.1 to 75.9 mg, depending on the concentration and volume nebulised. The HCQS solutions appear suitable for preclinical and clinical studies for potential COVID-19 treatment.
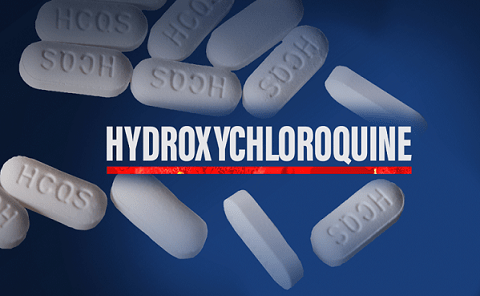
1. Introduction
Since December 2019, the world has been adversely affected by the coronavirus disease 2019 (COVID-19) pandemic, caused by severe acute respiratory syndrome coronavirus 2 (SARS-CoV-2). As of 17 July 2021, there were 189,482,312 confirmed cases globally, with 4,074,668 deaths. These tolls continue to increase daily at alarming rates. In addition to stressing public health systems, the pandemic has wreaked havoc on the economy and people’s livelihoods worldwide. Although various vaccines have been developed and inoculation programmes are progressively being launched in different countries, their effectiveness in achieving general population immunity and reducing viral transmission needs to be yet evaluated Vaccines may not completely restore the present situation to the pre-COVID-19 “norm” . In addition, the long-term safety of the vaccines is yet unclear due to the accelerated development of these products Public perception of the potential harm from the vaccines versus that from the infection will inevitably influence vaccination rate Furthermore, various vaccines have been reported to be less effective against SARS-CoV-2 variants, which has prompted questions on how the efficacy of future vaccines can be maintained to tackle incessant viral mutationsherefore, although vaccines are essential, their use alone may not be sufficient to solve the crisis. Drugs for treating the infection are required as a pragmatic strategy. The more effective drugs that are available, the better the health sector is equipped to combat this pandemic.
The course of COVID-19 progresses through two clinical phases. The early phase is predominated by viral replication, whereas the late phase features uncontrolled inflammatory or immune responses to the virus, leading to tissue damage. Thus, the mode of treatment for COVID-19 depends on the stage of the disease, with antiviral and anti-inflammatory therapies being more effective in the early and late phases, respectively. The United States National Institutes of Health advises using anti-SARS-CoV-2 monoclonal antibodies (casirivimab + imdevimab combination or sotrovimab alone) for non-hospitalised patients with mild to moderate COVID-19 at high risk of disease progression . Remdesivir is hitherto the only antiviral drug approved for treating COVID-19 by the United States Food and Drug Administration and European Medicines Agency . It is recommended for hospitalised patients on supplemental oxygen and can be used with dexamethasone if oxygen requirement is moderately high . For recently hospitalised patients requiring systemic inflammation using high-flow oxygen or non-invasive ventilation, baricitinib or tocilizumab can be added to dexamethasone with or without remdesivir. Dexamethasone is used alone in the most serious cases, when the patient requires invasive mechanical ventilation or extracorporeal membrane oxygenation In addition to the drugs mentioned above, the United States Food and Drug Administration has also issued emergency use authorisation for the bamlanivimab + etesevimab combination for mild to moderate COVID-19 . The American Society of Health-System Pharmacists has issued a comprehensive list of approved and experimental drugs for COVID-19 with their clinical evidence that is constantly updated Some of those drugs (e.g., hydroxychloroquine, azithromycin, lopinavir, ritonavir) have been or are being investigated for repurposing for COVID-19 In particular, hydroxychloroquine is an old 4-aminoquinoline antimalarial chemically similar to, but less toxic than, chloroquine . It has been employed for decades for treating autoimmune conditions such as rheumatoid arthritis and lupus erythematosus, due to its immunomodulatory effects . It is administered as hydroxychloroquine sulphate (HCQS) because this salt form is freely soluble in water (aqueous solubility of 1 in 5), with 1 mg of HCQS being equivalent to about 0.775 mg of the baseAbsorption from the gastrointestinal tract is rapid and extensiveThen, it undergoes hepatic first pass metabolism and results in an oral bioavailability of 79% [
The proposed use of hydroxychloroquine to prevent and treat COVID-19 is based on its antiviral and immunomodulatory effects reported in the literature. Hydroxychloroquine and chloroquine showed in vitro antiviral activity against SARS-CoV-2 before and after infection in Vero cells, which were derived from the kidney epithelial cells isolated from an African green monkey. When the cells were pre-treated with the drugs for 2 h before infection, the half maximal effective concentration (EC50) of hydroxychloroquine and chloroquine for inhibiting viral replication after 48 h of incubation was 5.85 and 18.01 µM, respectively On the other hand, their EC50 was 0.72 and 5.47 µM, respectively, when they were added after infecting with the virus at a multiplicity of infection (MOI) of 0.01. In another study, their EC50 on Vero E6 cells (ATCC-1586) at the same MOI was 4.51 and 2.71 µM, respectively The different EC50 for both drugs between the two studies might be due to the different Vero cell lineages used. Nevertheless, those levels were not lethal to the cells because they were significantly lower than the half-maximal cytotoxic concentrations (CC50) on Vero E6 cells (249.50 and 273.20 µM for hydroxychloroquine and chloroquine, respectively)From these in vitro data, hydroxychloroquine and chloroquine may potentially be used for the prophylaxis and treatment of COVID-19.
Although the antiviral mechanism of these drugs is unclear, they have been shown to prevent the attachment of SARS-CoV-2 to angiotensin-converting enzyme 2 (ACE-2), sialic acid-containing glycoproteins, and gangliosides on the surface of host cells to which the virus needs to bind for entry . In addition, the drugs are weak bases so they increase the pH of the normally acidic endosomes and lysosomes in host cells The alkalinisation alters the homoeostasis of these intracellular organelles and hinders various processes in the viral life cycle tha
Materials and Methods
HCQS powder of United States Pharmacopoeia (USP) grade (Lot 1910P031, Batch 033600-192021) was purchased from Sci Pharmtech Inc. (Taoyuan, Taiwan). Chromatographic grade methanol and acetonitrile were bought from RCI Labscan (Bangkok, Thailand) and Honeywell (Morris Plains, NJ, USA), respectively. Deionised water was obtained from a MODULAB® High Flow Water Purification System (Evoqua Water Technologies, Pittsburgh, PA, USA).
Isotonic and pH-neutral solutions containing 20, 50, and 100 mg/mL of HCQS were prepared in volumetric flasks. Then, they were transferred to 50 mL of polypropylene centrifuge tubes (Corning, Corning, NY, USA) and stored in darkness at ambient temperature until use. The osmolality of the solutions was measured with a K-7000 vapor pressure osmometer (Knauer, Berlin, Germany). The cell and head temperatures were set as 60 °C and 62 °C, respectively, and allowed to stabilise for an hour before use. These temperatures followed those recommended in the instrument manual for calibrating and measuring sodium chloride aqueous solutions . The measurement time and gain were 1.5 min and 16, respectively. Approximately 1 mL of each sample solution was drawn into glass microsyringes and inserted into the osmometer. One droplet from each sample was dispensed onto the thermistor for each osmolality measurement. The droplet was replaced by a new one when repeating the measurement. The experiments were conducted in quadruplicate (n = 4) for each HCQS solution. The target osmolality range was 260–360 mOsmol/kg H2O . The pH of the solutions was measured with a pH 700 benchtop meter (Oakton, Vernon Hills, IL, USA). The target pH range was 6.8–7.5
Results
Two batches of 100 mg/mL solutions were made (Batches A and B). Batch A was used to obtain the 20 mg/mL solution by dilution, while Batch B was used directly for the 100 mg/mL experiments and for making the 50 mg/mL solution. No drug degradation was observed over the 15 days during which all the experiments were performed. The drug concentration, osmolality, and pH of the HCQS solutions before and after filtration are presented
he osmolality and pH of all solutions were within the target ranges, regardless of filtration. The five-fold dilution of the Batch A 100 mg/mL solution to 20 mg/mL reduced the osmolality from 323.0 to 286.5 mOsmol/kg H2O, but it was still within the target range. HCQS concentration was not affected by filtration. On the other hand, osmolality and pH decreased after filtration, but the difference was not significant. Similar trends were observed for the Batch B 100 mg/mL and 50 mg/mL solutions. The osmolality and pH of the 50 mg/mL were between those of the 20 mg/mL and 100 mg/mL solutions.
The retention time of the HCQS peak in the HPLC chromatogram was about 8 min. Table 2 shows the regression equations of the calibration curves with the mean slopes and y-intercept. They were obtained using fresh standard solutions over 11 and 14 days with deionised water and 50:50 v/v methanol/water as the diluent, respectively. The standard curves were similar between the days and were linear (r2 ≈ 1) from 6.25 to 1000 µg/mL. The detection and quantitation limits were derived by Equations (3) and (4), respectively [55]. The values of slope featured in these equations were taken to be the mean slopes shown in Table 2.

Discussion
The ClinicalTrials.gov (accessed on 20 May 2021) database records several clinical studies on inhaled hydroxychloroquine for COVID-19 at various stages of progress, from recently completed to not yet recruiting [58]. There are Phase 1 safety, tolerability, and pharmacokinetic studies on healthy subjects (ClinicalTrials.gov Identifier: NCT04461353, NCT04497519, NCT04697654), as well as efficacy studies on COVID-19 patients (NCT04477083, NCT04731051), but no results from the completed trials were available as of 17 July 2021. The formulations investigated included nebulised solutions (NCT04461353, NCT04731051), nebulised liposomal suspension (NCT04697654), and inhaled dry powders (NCT04497519, NCT04477083).
Conclusions
Inhalable droplets of isotonic and pH-neutral HCQS solutions generated from vibrating mesh nebulisers were characterised. Droplet size decreased with increasing solute concentration. A range of emitted and fine particle doses were obtained with 20–100 mg/mL HCQS, with the 100 mg/mL solution potentially able to achieve sufficiently high drug concentrations in the airways for antiviral effects of COVID-19 with low systemic absorption.
Credited to Waiting Tai
Comments